Flex-Lock: Ride Like a Soft Booter. Tour Like a Hard Booter.
Imagine touring up a hard packed skin track on your splitboard: your legs are on fire as you spit and fight with every ounce of strength and focus just to keep your edges griped to the slope. You’re trying to keep your cool so your buddy on AT gear, who’s effortlessly dancing up the path, doesn’t notice your struggle. Your downhill leg is contorted and awkwardly twisted inward as you desperately try to keep your edges from rolling down hill and breaking free from the firm snow. You even resort to the karate chop technique to set your downhill foot. When you do make it to the top, you realize that you’re physically worked because of the incredible effort you just exerted. This was the story of a soft boot splitboarder until now.
Earlier this summer we sent out an instagram video releasing our patent-pending Flex-Lock, available November 2015, with a very strong statement: Ride Like a Soft Booter. Tour Like a Hard Booter. We know this is a strong statement, and based on our 3 seasons of experience using versions of the Flex-Lock we know it’s true. Qualitative experience is good, but we wanted to know more; we wanted hard evidence to show how much better the Flex-Lock is compared to an elastomer ski strap or velcro power strap around the top of your boot and highback and we wanted to know how it stacked up against the gold standard for touring performance, the AT boot. We thought you would too. So we set up an experiment to measure the relative edging performance of these boot/binding combinations. The baseline is a splitboard binding without any lateral support and each configuration is measured as an improvement over this baseline; the greater the percentage increase the better the edging performance. Here are the results:
As shown in the chart above, the ski strap and power strap give a small 15% increase in edging performance over a binding without lateral support. The Flex-Lock gives a splitboarder a 101% increase in edging performance compared to a binding without lateral support, which is on par with an AT boot at 104% increase. These results are pretty definitive. By adding the Flex-Lock to your Karakoram Splitboard Bindings you will experience the edge gripping power that AT skiers have enjoyed for years. It’s your turn to dance up the hill and still have the freedom to ride with the flip of a lever- Ride like a soft booter. Tour like a hard booter.
For more information on the biomechanics of the Flex-Lock and details on the edge force transfer test continue reading below.
Biomechanics of the FlexLock:
Because of the intrinsic mechanics of the ankle joint, our downhill “split ski” is always on a disadvantaged mission to literally “roll” off the slope. The uphill ski is almost never a problem because during splitboard touring, the ankle joint is exceptionally powerful in what biomechanics researchers call “eccentric eversion”. Put another way, you can’t “roll” your ankle outward past just a few degrees of motion (3). On the contrary, ankle “inversion” (tendency to roll one’s ankle inward) is actually quite easily done because of the drastically larger range of motion in that direction (3). These differences in range of motion are almost entirely due to the skeletal and ligament structures of the ankle joint. However, when it comes to avoiding extreme ankle eversion, there are also significant neuromuscular contributions. To elaborate briefly here, the muscles that are responsible for ankle “inversion” (classic rolled ankle motion) tend to be at a mechanical advantage in comparison to the muscles that “evert” the ankle (2,3). Therefore, your uphill ankle while side-hill traversing can easily combat the forces that want to “evert” the ankle off the slope because of its skeletal and neuromuscular advantages in that direction of motion.
Based on these mechanical characteristics of the ankle joint, the question then becomes how are soft-boot splitboarders able to create edge pressure on their downhill ski at all? As most of us know, the human body is amazing at adaptation. If the ankle joint is weak in inversion, and you need to create a downward medial force (inside ski edge pressure) the natural tendency is therefore to begin to rely on the knee joint. Imagine a “knock kneed” position, except that only the downhill leg is doing this. In biomechanics, this position is called “valgus knee alignment”. The opposite (bow-legged) is called “varus knee alignment”. It turns out that by creating an “extreme valgus” at the knee (exaggerated knock knee) a soft-boot splitboarder is able to increase inside medial edge pressure. The problem with this is that it is extremely inefficient and uses up a tremendous amount of cellular energy. For example, when the knee is in extreme valgus, the hip joint is negatively impacted and the athlete is not able to optimally activate the primary muscles of locomotion: The gluteal extensors (8). They therefore rely on smaller muscles, and fatigue faster (3,11). Furthermore, its exceptionally unhealthy for the knee itself. Exaggerated valgus increases the chances of tearing or rupturing the Anterior Cruciate Ligament (ACL), cartilage tears, medial collateral ligament tears (MCL), as well as opens the door for Patellofemoral tracking problems, which can lead to kneecap dislocations, arthritis, and chronic pain (4,5).
Where the puzzle becomes more complex, is that most of these motions at the ankle, knee and hip are actually very important for snowboarding itself, and aren’t always damaging to the joint when part of a balanced kinetic chain. The act of “riding” a snowboard brings forth a completely different set of biomechanical needs in comparison to uphill travel. For example, it’s critical that we maintain and optimize the ability of the ankle to move through a relatively large range of motion in both inversion and eversion, as well as for the knee be able to create “valgus moments”. Being able to move into and out of these body positions are an integral part of what gives soft-boot snowboarding such a tremendous degree of finesse and control over the snowboard, and what allows snowboarders to respond to undulations and subtleties in terrain to such a high degree (1,6,7,8).
With a good understanding of the biomechanical needs of splitboarding, the question for us therefore became “How do we rapidly and efficiently lock and unlock medial flex to optimize both climbing AND riding?” We knew about various other means of medio-lateral stiffness devices for touring including rubber ski straps and velcro power straps around the top of the boot, but we were unsatisfied with the side hilling performance these solutions provided. We still found ourselves utilizing this “extreme valgus” to pressure the edge, and the ease of use in the field was cumbersome and time consuming to implement. We hypothesized that by triangulating a force vector off the ankle ratchet to the medial top of the highback, we would completely eliminate any rotational moments of torque about the highback, and would also not interfere with the entry and exit of the foot in the binding. Because we would be relying on the “side bending” properties of the highback and aluminum binding chassis, and not the torsional characteristics of the highback (highbacks are designed to twist naturally) we would be able to achieve tremendously greater stiffness in the medial compartment.
We first prototyped the FlexLock 3 seasons ago with a hodgepodge of old strap components, and discovered almost immediately that on a qualitative level, we were really on to something. The ankle roll and extreme knee valgus discussed above was almost completely eliminated, we had far better edge traction on firm snow, and we found that steep hill climbs no longer required as much physical exertion because of the improvement in our gait biomechanics.
It’s one thing to have a “feeling” that something works well, or is better than other options, but opinions don’t mean anything without hard evidence. We wanted to know just how much of an improvement in edge force transfer this concept offered with true, quantifiable data. And not just that, we wanted to know how the Flex-Lock stacked up to the gold standard for side hill touring, a 4-buckle AT plastic freeride ski boot without modifications for splitboarding. We thought you’d like to know this as well.
METHODS:
To answer this question we developed a simple edge force transfer test to measure the force transferred from the boot/binding combination at a given angle of deflection (mimicking knee valgus) through the edge of the ski.
Test configurations were as follows:
- Binding Only: no lateral stiffness device, Karakoram Prime1 binding with Flex-Lock off with old Burton Ion. **
- Elastomer Ski Strap: Karakoram Prime1 binding with Elastomer Ski Strap around highback and upper boot cuff on old Burton Ion. *,**
- Velcro Power Strap: Karakoram Prime1 binding with Garmont Velcro “Power Strap” on old Burton Ion. *,**
- Flex-Lock: Karakoram Prime1 binding with FlexLock with old Burton Ion. 2
- AT Boot: Garmont 4-buckle hard plastic ski boot on a Dynafit Tour Mode with power strap engaged (gold standard).
* Both the Ski Strap and Garmont Power Strap were cinched as tight around the boot and highback as tight as we could get them. Each of the 4 buckles on the Garmont boot were also adjusted to be as tight as possible.
** All soft boot configurations used the same boot a size 8 burton Ion, and same Prime1 binding. The straps were the same tightness for all tests (never released or tightened during the test)
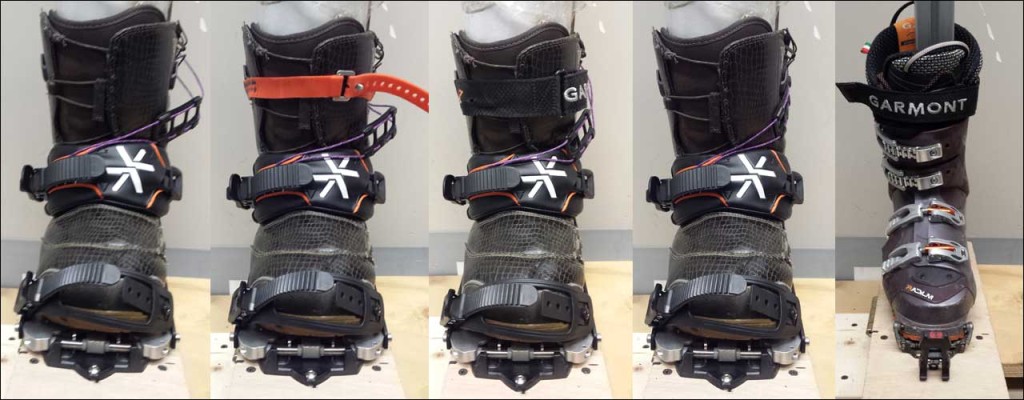
Figure 1- Test Configurations from left to right: Binding only, Elastomer Ski Strap, Velcro Power strap, Flex-Lock, AT Boot
Test Setup:
The simple edge force transfer setup shown below has a tour mode interface attached to a “ski” which in our case is a ¾” piece of plywood with t-nuts for mounting. The ski is attached to a lever that extends to the center of a digital scale. The lever is connected on the outside ski edge side by a hinge allowing it to rotate towards the scale. A 1 meter aluminum extrusion “leg” extends up from an aluminum foot inside the boot. The “leg” is wrapped to increase the diameter from the ankle up to approximate the diameter of a leg in the boot. A pointer is secured to the top of the “leg”. Tick marks are made on the wall for the desired angles of deflection.
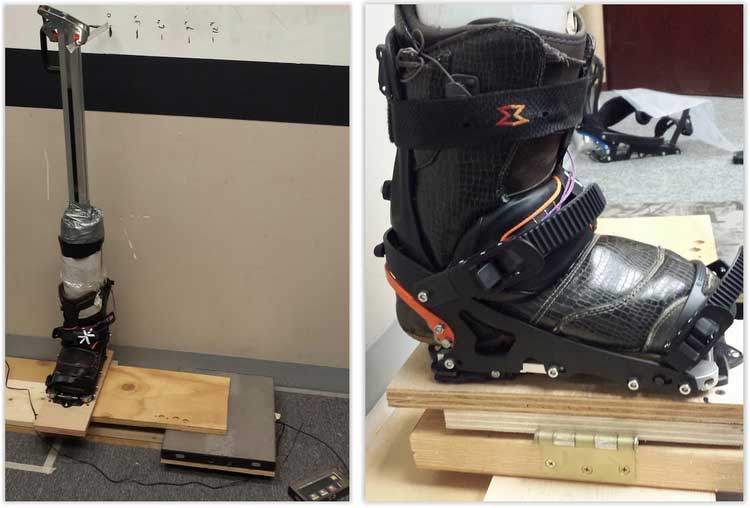
Figure 2-The desired configuration is mounted on the tour mode bracket and the lateral stiffness device is attached per each configuration and the aluminum leg is deflected to given angles (valgus) and the force is measured on the digital force plate. The force plate is allowed to stabilize and the force reading is recorded. This is repeated for each configuration to each deflection angle 4 different times, and averaged to account for tester variation.
Test Protocol:
- Setup configuration on tester
- Return aluminum leg so pointer is at zero tick mark at rest
- Deflect leg so pointer is centered on first angle mark and hold until scale stabilizes
- Record scale measurement
- Continue deflection to the next three tick marks repeating steps 3 and 4 at each mark
- Repeat steps 2-5 for 4x runs per configuration
- repeat for all configurations
In this test setup the absolute measured force in each configuration is an arbitrary number, as the force is proportional to the length of the lever going to the scale and is not representative of real world “on snow” forces. Because this study is looking specifically at relative differences in force transmission between test configurations and not absolute measured edge force, the recorded values for each scenario are used solely for statistical comparison.
Our force plate is a large stainless steel rectangular (15” x 12”) single axis (Z only) shipping scale manufactured by “JSHIP”. It is not a not a certified “biomechanics force plate”; however as stated, we were only measuring relative force differences. In order to maximize testing sensitivity, we set up a 20 inch piece of plywood to act as the lower moment arm. This allowed us to transmit force from the binding / ski pivot directly into the center of the scale, and thus equally distributed pressure to all 4 of its sensors (one at each corner).
RESULTS:
Figures 1 and 2 shows the Ski strap and the PowerStrap as approximately a 15% increase in force transfer to the edge over the binding alone. The Flex-Lock and AT Boot both show approximately a 100% increase in force transfer to the force plate over the binding alone.
Figure 5 displays the same data, but normalized such that the “Binding Only” configuration is the baseline. All other configurations are represented as improvement in force transmission over this baseline. Again, the Ski Strap and Power Strap configurations show approximately the same degree of force transfer. The difference in force transfer under any given angle of valgus between the Plastic AT boot and the FlexLock configurations is 2.88%.
DISCUSSION:
In the elastomer Ski Strap and Velcro Strap configurations, the connection is only to the top of the highback. Forces from the Valgus position cause the Ski Strap and Velcro Strap to twist the highback significantly before the loads are transferred to the edge (see figure 6 below). Highbacks are designed to twist in the transverse plane in order to allow for the proper biomechanics of riding. Therefore, relying on the rotational characteristics of the highback is highly inefficient when attempting to transfer a linear medial force to the ski edge, as the improvement in load transfer to the edge is limited by the torsional stiffness of the highback. The data supports this hypothesis, as the both strap configurations only increased medial force transmission by approximately 15% over the “Binding Only” configuration.
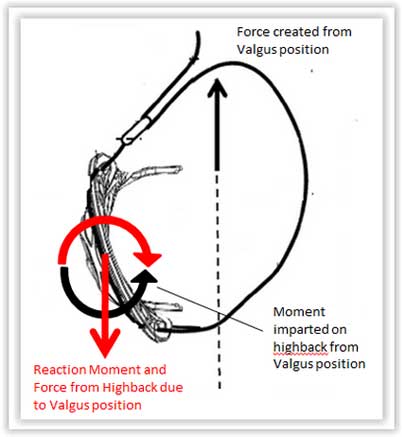
Figure 6- Third Strap configurations force and moment free body diagram showing large moment imparted on the highback.
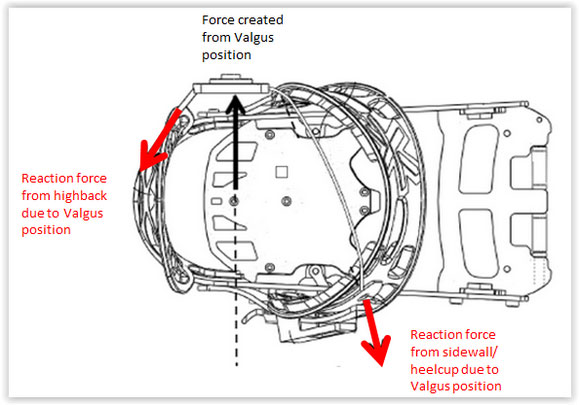
Figure 7- Flex-Lock free body diagram showing reaction forces on highback and sidewall/heelcup cancelling each other out.
Mechanically, the Flex-Lock functions in a completely different manner than the elastomer Ski Strap and Velcro Power Strap. The Flex-Lock connects from the top of the medial side of the highback diagonally across the top of the boot and directly to the sidewall/heelcup. This configuration allows the force from the Valgus position to be reacted directly by both the highback and sidewall/heelcup, see figure 7. Additionally the majority of the load is transferred to the highback in bending as opposed to torsion. The highback is dramatically stiffer in frontal plane bending than it is in torsion. This bending load resistance in conjunction with the load being reacted at two locations: The highback and sidewall/heelcup, allows the Flex-Lock to optimally transfer the side load from the Valgus position to the edge of the ski in a manner unobtainable by configurations relying on the torsional stiffness of the highback. The results of the edge force transfer test validate this with a 100+% increase in force transfer over the “Binding Only” and an 85% increase over the ski strap and power strap configurations. The Flex-Lock also proves to be in the same category of force transfer to the ski edge as the gold standard AT boot, with a nominal difference of 2.88%.
The critical information to take from this study, is the potential impact of the FlexLock system on the biomechanics of splitboard touring. The biggest downfall to all soft-boot riders in the backcountry is sidehilling capability, and the extreme negative impact on efficiency that exaggerated valgus positioning has. We were able to show a dramatic improvement in side load force transfer at any given degree of valgus positioning at the knee. At each point of valgus deflection, we literally recorded a 2X increase in forces measured at the scale. What this means in a real world application is that in order to adequately pressure the ski edge, you won’t need to create this “dramatic valgus angle” at the knee. One of the most common complaints by most experienced splitboarders is that on long, firm snow sidehill traverses, they often find themselves “unstrapping from the binding, and recentering the foot in order to regain edging performance”. This is due to the fact that a snowboard boot will literally rotate within the binding itself over long distance side-hilling travel, and the valgus position begins to progressively lose its impact on increasing linear edge pressure; therefore requiring progressively more and more valgus loading at the knee. When you are able to reduce the reliance on valgus positioning such that your lower extremity displays “normal” gait biomechanics while climbing, you’re not only increasing efficiency, but you are also decreasing the amount of wear and tear on the joint(4,5).
Its also worth noting that the FlexLock may have a particular benefit to female splitboarders. In the world of orthopedics, it is very well documented that female athletes are in general at about a 75% greater likelihood of suffering an ACL injury in comparison to their male counterparts (4,5). There are wide ranging theories about why this is the case, but the general consensus in the literature is that a greater width in the pelvis, greater femoral anteversion, sex-related differences in femoral intercondylar notch width, as well as increased Q-angle all lead to a far greater degree of overall valgus alignment in the lower extremity (10). Although not addressed in this study, we hypothesize that female splitboarders would tend to display an even greater degree of exaggerated valgus during sidehill climbing on a splitboard. They could therefore be at a higher risk of injury, and have their gait efficiency even more negatively impacted than male splitboarders. The FlexLock therefore may offer protection, or at least assist in the reduction of valgus alignment during sidehill touring.
Another area of research we would like to investigate on the FlexLock, would be its impact on long flat travel, or just “normal touring” in soft boots. Although the literature on splitboarding biomechanics is essentially non-existent as of 2015, it has been our qualitative experience that those individuals who display intrinsic valgus alignment tend to have a harder time “tracking” in a straight line on long, flat terrain; particularly if the snow is firm. We would therefore like to do a quantitative lower extremity study on the impact of the FlexLock on gait biomechanics in “normal touring”. Furthermore, it would be interesting to research the physiological impacts of reduced valgus positioning, and see if there are any improvements in metabolic function. A study in 2011 by Duc and Cassirame did a comprehensive analysis on the physiology of ski mountaineering racing (9). It would therefore be interesting to gather similar data in splitboarding, and see if there are any meaningful impacts on metabolic efficiency.
Like all scientific investigations, there are limitations to any study. We recorded each measure at each angle of deflection a total of 4 different times (16 data points for each configuration) and then averaged those numbers in order to minimize tester error. In order to achieve a higher degree of statistical power and further decrease testing error and / or bias, we could have recorded and averaged more data points at each point of valgus, and also had more than one person doing the measurements. It’s difficult to know if this would have elicited different results, because the level of improvement between baseline and with “FlexLock On”, and “Gold Standard AT Boot” (total of 100+% increase), we feel a larger sample size of data points, and using a larger number of testers, simply would have been redundant and brought about similar results. It’s worth noting that more precise angular measurement devices could have been used. Also our force plate is a generic stainless steel shipping scale with only one axis of measure (Z-axis). Our test setup was also made of wood, and the point of rotation was a simple “door hinge”. Further, both the Karakoram and Dynafit tour modes were mounted to a “plywood ski” via T-nuts. These factors thus could have altered the force numbers in relation to a real world application, but again, this study was set up to be relative between configurations and each test was compared to the previous on the same testing jig. In our testing setup with the aluminum extrusion coming out of the boot, the ski boot was able to be cinched tighter around the rod than the snowboard boot was, which could have reduced the force numbers from all snowboard boot configurations. It’s also highly probable that because there is no “ankle joint” or “neuromuscular contribution” in the test, the results may have been different had a true biomechanics prosthetic leg or cadaver leg been implemented; However even in those two options there still would not be a “muscular” component to the test. Further, we only used a single splitboard binding (Karakoram Prime1) and a single boot (Old Burton Ion). It would be interesting to see the differences in the same test across different binding and boot models.
On that note, we also only used a single AT boot model (4 Buckle Garmont freeride boot with Power Strap). Taking measures on other “Gold Standard” models may bring about different results based on boot stiffness, buckle position, and even plastic boot modifications for splitboarding. Many hard booters cut down the medial portion of their boots in order to increase medial flex, and we hypothesize that this could significantly lower the degree of medial force transfer at all valgus angles, potentially even lowering it below that of the FlexLock system. We want to note that most AT skiers tour with their boot buckles either wide open, or significantly looser than while downhill skiing. This test was done on the boot while it was cinched as tight as possible across all 4 buckles and with the power strap engaged at full tightness. In that configuration, the “Gold Standard” was only 2.88% more efficient at transferring a load into the medial force plate than the FlexLock. It could therefore be postulated that doing this test on a less stiff boot with all buckles and power strap wide open, and which is significantly modified for splitboarding, could bring the medial stiffness significantly below that of the FlexLock. More investigation is needed however.
All in all, the results of this study have a high degree of application to splitboard touring performance. If you want to improve your sidehill edging by approximately 15%, you can use an elastomer Ski Strap or a Velcro Power Strap. But if you truly want to sidehill with the efficiency of a hardbooter, but still ride in soft snowboard boots, utilize the FlexLock System. With the flip of a lever you can have AT boot support, flip the lever back and you can surf down the hill in your favorite snowboard boots. Tour like a hard booter and ride like a soft booter with the Karakoram Flex-Lock.
For more information on the Flex-Lock or to buy a set: https://www.splitboardbindings.com/products/flex-lock/
Bibliography
- Alexander, M. (n.d.). Kinematics of the Ankle Joint Complex in Snowboarding. Yearbook of Sports Medicine, 18-19.
- Enoka, R., & Enoka, R. (2002). Neuromechanics of human movement(3rd ed.). Champaign, IL: Human Kinetics.
- Hamill, J., & Knutzen, K. (2003). Biomechanical basis of human movement (2nd ed.). Philadelphia: Lippincott Williams & Wilkins.
- Hewett, T. (2005). Biomechanical Measures of Neuromuscular Control and Valgus Loading of the Knee Predict Anterior Cruciate Ligament Injury Risk in Female Athletes: A Prospective Study. American Journal of Sports Medicine, 492-501.
- Hewett, T., Myer, G., Ford, K., Heidt, R., Colosimo, A., Mclean, S., . . . Succop, P. (n.d.). Neuromuscular Control and Valgus Loading of the Knee Predict ACL Injury Risk in Female Athletes. Medicine & Science in Sports & Exercise.
- Hirose, K., Doki, H., & Kondo, A. (n.d.). Dynamic motion analysis of snowboard turns by the measurement of motion and reaction force from snow surface. Procedia Engineering, 754-759.
- Hirose, K., Doki, H., & Kondo, A. (n.d.). A Study on the Dynamic Motion Analysis of Snowboard Turns by the Measurement of Gliding on the Actual Snow Field. Trans.JSME(C) Trans.JSME Ser.C Transactions of the Japan Society of Mechanical Engeneers,Series C Trans.JSME, C Trans.JSME, Ser.C Trans.JSME C TRANSACTIONS OF THE JAPAN SOCIETY OF MECHANICAL ENGINEERS Series C, 897-907.
- Krüger, A., & Edelmann-Nusser, J. (n.d.). Biomechanical analysis in freestyle snowboarding: Application of a full-body inertial measurement system and a bilateral insole measurement system. Sports Technol. Sports Technology, 17-23.
- Duc, S., Cassirame, J., & Durand, F. (2011). Physiology of Ski Mountaineering Racing. International Journal of Sports Medicine Int J Sports Med, 856-863.
- Lewis, T. (n.d.). Anterior Cruciate Ligament Injury in Female Athletes: Why are women so vulnerable? Physiotherapy, 464-472.
- Mauntel, T., Begalle, R., Cram, T., Frank, B., Hirth, C., Blackburn, T., & Padua, D. (n.d.). The Effects of Lower Extremity Muscle Activation and Passive Range of Motion on Single Leg Squat Performance. Journal of Strength and Conditioning Research, 1813-1823.
[…] For more details on the edge force transfer test experiment and how the Flex-Lock is able to out-perform “third straps” including the full biomechanics behind the Flex-Lock check out our full blog post here. […]
[…] Flex lock is an add on to the Karakorum binding system that increases rigidity when doing something like sidehilling. Sidehilling is what most splitters complain about, and why most jump to a hard boot setup, so if this works as advertised, then holy smokes is that incredible. They wrote a blog post detailing some experiments they ran and came up with results that are pretty … […]
[…] Flex lock is an add on to the Karakorum binding system that increases rigidity when doing something like sidehilling. Sidehilling is what most splitters complain about, and why most jump to a hard boot setup, so if this works as advertised, then holy smokes is that incredible. They wrote a blog post detailing some experiments they ran and came up with results that are pretty … […]